Key Points
- Dr. Shapiro’s work combines the physical interactions of ultrasound with synthetic biology – the field of science that redesigns organisms for useful purposes.
- At Caltech, Dr. Shapiro leads a 28-person research laboratory dedicated to ultrasound imaging and focused ultrasound.
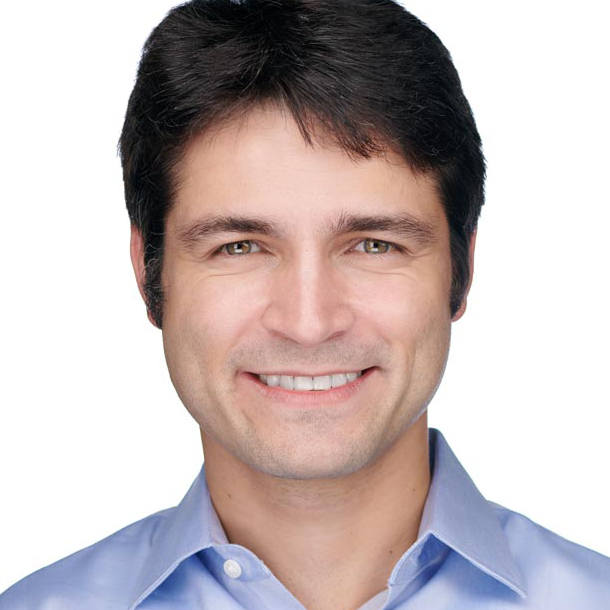
Mikhail Shapiro, PhD, is a new type of scientist, one who combines the physical interactions of ultrasound with synthetic biology—the field of science that redesigns organisms for useful purposes by engineering them to have new abilities. Besides being a Professor of Chemical Engineering, a Heritage Medical Research Institute Principal Investigator, and the Director of the Center for Molecular and Cellular Medicine at the California Institute of Technology (Caltech), Dr. Shapiro has a 28-person research laboratory dedicated to ultrasound imaging and focused ultrasound. We interviewed him to learn about his incredible work creating bidirectional communication with cells by engineering them to express gas vesicles, making them sensitive to temperature changes, and modulating their activity through acoustically targeted receptors.
Focused Ultrasound Work
When and how did you get interested in focused ultrasound?
Ultrasound imaging was my starting point, but over time our lab has developed several major efforts in focused ultrasound perturbation of cells. The first time that I noticed focused ultrasound was when I was in graduate school at the Massachusetts Institute of Technology (MIT). I was working on MRI imaging agents. Kullervo Hynynen was still in Boston at that time, and I heard him or one of his colleagues talk about using ultrasound to open the blood-brain barrier. I thought that it sounded totally crazy and wanted to learn more. After I saw his beautiful results, I was completely hooked, but I couldn’t add it to my research until I started my lab at Caltech in 2014. But then we started working on focused ultrasound right away.
What are your areas of interest in focused ultrasound?
Creating bidirectional communication with cells is the most important part of our focused ultrasound research. We study how focused ultrasound affects cellular function, use it to control cellular function, and create a feedback loop to make adjustments. Besides observing the effects of the focused ultrasound on cells, I also wanted to perturb them for basic biology research and to treat cells for medical purposes.
This is how we use focused ultrasound differently than most other researchers – we are not using the effects of ultrasound by themselves; we are using ultrasound in the service of cell-based and gene-based therapy. I believe that the future of medicine is the injected or ingested cell-based therapies that travel to the specific sites of disease, survey the molecular environment, make intelligent diagnoses, and then take action based on those assessments. These things are already happening in cancer immunotherapy with engineered T-cells and FDA-approved gene therapies for rare diseases. These cells and genes can be much more specific in what they do compared to a simple molecule. They can also be more specific and selective in what they do compared with “brute force” ablation.
What we are trying to do is add the ability for spatial control and communication with these cells – to see where they are and what they are doing and tell them what do to.
How do you see the cells that you are perturbing?
We take advantage of the same genetic engineering that makes cell-based therapies possible. Most of these cells, such as CAR-T cells in cancer immunotherapy, have been genetically modified to have receptors that recognize tumors. Similarly, we developed a method to genetically modify cells so that they can be imaged and controlled by ultrasound. For this purpose, we adapted some very unusual air-filled proteins, called gas vesicles, which originally evolved as flotation devices in aquatic photosynthetic microbes, as imaging agents for ultrasound. The gas vesicles are genetically encoded, so we can put these specific genes into bacterial or mammalian cells. Based on the gene circuit we implement, the cells will produce gas vesicles when they encounter a certain signal in their environment. We can then use ultrasound imaging to see the gas vesicles that the cells are producing and see that the cells have been activated. And more recently we discovered that we could use focused ultrasound to “blow up” those gas vesicles and cause local effects like drug release or localized mechanical damage by seeding cavitation. It is like local production of a warhead that can be remotely triggered.
But it does not always have to be so violent. The other mechanism that we are developing is the ability for cells to sense small changes in temperature and use that as an input for activating a therapeutic function. In this mode, focused ultrasound is used at a relatively low intensity regime to heat the tissue at the desired focal zone, within the range of 37 to 42 degrees Celsius, which is a range that most tissues tolerate. The cells then respond to the change in temperature because they contain temperature-sensing gene circuits that can convert the information into the activity of our choice (e.g., expression and local secretion of a protein or a therapeutic; or turning on the ability to recognize and kill nearby cells via engineered T-cell receptors). Having the ability to plug in to genes and protein circuits inside cells gives us a wide range of capabilities. We are making the link between cellular engineering and ultrasound – both for imaging and for control.
We are not just using focused ultrasound for ablation; we are using it as a cellular command signal.
How do you explain your interest in so many areas of research?
We are less disjointed than it might seem, because there is a common core, or intersection, between ultrasound and synthetic biology. Everything grows around that. My undergraduate degree was in neuroscience, so I was a biologist before becoming an engineer. I enjoy combining these disciplines. Understanding what is happening in the brain requires an understanding of its cellular functions. The central processing unit is the neuron, which is a type of cell. This motivated my original search for technologies that can talk to cells deep inside the body.
What diseases or clinical indications do you study?
We look for indications where our technology can have an impact. We have some projects that are feasible in the near-term and others that are more fundamentally challenging that we are working on for the long-term.
For cancer, we are interested in developing treatments for primary tumors and metastases that can be identified, visualized, and accessed by ultrasound. For example, brain cancers are usually formed by lethal primary masses. We are also collaborating on an ovarian cancer project. Ovarian cancer usually involves several masses that may be benign or malignant, difficult to biopsy, and challenging to remove surgically.
We are also interested in the brain, and about a third of our lab is working on neuroscience projects. We are developing ultrasound as a tool for neuroscience research and brain treatments. On the treatment side, I am excited about our work in acoustically targeted chemogenetics. Our 2018 paper in Nature Biomedical Engineering showed how we can use ultrasound to open the BBB to allow systemically administered viral vectors to introduce chemogenetic receptors into neurons in the brain. After they are expressed, these chemogenetic receptors make the neurons at the ultrasound-targeted site become excited or inhibited when we administer a simple pill. Instead of responding to neurotransmitters like normal receptors, the chemogenetic receptors respond to otherwise inert small molecule drugs that are ingested and are permeable across the BBB (no additional BBB opening is required).
There is a one-time ultrasound treatment to open the BBB and allow the viral vectors encoding chemogenetic receptors to enter a specific region of the brain—or brain circuit. After waiting a few weeks for the expression of the receptors, a pill is administered whenever we want to turn the functionality of the targeted brain circuit up or down. This is a type of selective pharmacology. While this might seem futuristic, it is based on well-established components. BBB opening and chemogenetics have been around for some time, and the type of viral vector that we used in the 2018 Nature Biomedical Engineering paper has been approved by the FDA for certain indications.
The 2018 study in mice focused on the hippocampus; we were able to prevent mice from forming memories on demand—or turn off memory formation. Based on that work, our laboratory received a grant from the NIH BRAIN Initiative to scale this technology to nonhuman primates. We are also improving each aspect of the technology, including the viral vectors. For example, we are modifying the amino acid sequence of the viral capsid to improve its entry into the brain across the BBB.
For neural imaging, we are using the technology developed by Mickäel Tanter, PhD, in Paris, for studying hemodynamic brain activity changes. We have a collaboration with Richard Anderson, PhD, a scientist who works on brain-machine interfaces here at Caltech, to use the functional ultrasound readouts from the brain as a brain-machine interface. The results of this work, just published in Neuron, have amazed me because they showed that the information content ultrasound can extract from the brain are sufficient to predict intended movements before they happen. We have been doing those experiments in non-human primates and have recently started a study in humans.
For molecular imaging of brain activity, we are trying to engineer our gas vesicles to produce an ultrasound signal that depends on molecular events in neurons, such as changes in gene expression or the activity of certain proteins such as enzymes. This is similar to what other scientists are doing with fluorescent biosensors, which are well established but are limited by the depth of the neurons relative to how far light can penetrate. Therefore, if we can express our gas vesicle-based sensors in the brain, we can look at the signals with ultrasound instead of light. This technology could answer basic neuroscience questions, such as, “How does emotion work? Or how do we recognize faces?”
What types of brain diseases or disorders might the chemogenetic technology work for?
Neurological diseases and mental health disorders—anywhere today’s surgeons are inserting electrodes for deep brain stimulation (DBS) or doing ablation. This might include Parkinson’s disease, obsessive-compulsive disorder, epilepsy, depression, and more. I think this technology has the potential to provide an alternative to DBS because focused ultrasound provides spatial targeting, and it would be easier for patients to take a pill instead of undergoing invasive surgery. The physicians could adjust the dosing (amount and interval) of the pharmacology. The key is that this technology makes the drugs only active in the targeted regions.
What is the overall goal of your work?
Our overall goal is to enable noninvasive imaging and control of cellular function.
We are designing tools and then determining which biological or medical problems they might solve, but it is a two-way street. I am a biochemical engineer who develops technologies, but I want to be sure that the technologies that I develop are useful. Before my team and I started working on this, few other scientists—as far as I know—had connected proteins so directly to ultrasound. It is a wonderful intellectual challenge to solve, and how far we can push this technology is our primary driver.
Many postdoctoral researchers who come to work in my lab are ultrasound experts who want to work on cellular function. We are building a good track record of turning ultrasound engineers into people who are able to also work on synthetic biology. Now several of them are starting their own labs.
Are you creating a new field between ultrasound and cellular biology?
I hope so.
What are your funding sources?
Our major past and current research funding has come from the following sources:
- The National Institutes of Health (NIH), including awards from the National Institute of Biomedical Imaging and Bioengineering (NIBIB), the National Institute of Neurological Diseases and Stroke (NINDS), the National Institute of Mental Health (NIMH), and the National Cancer Institute (NCI). Our NINDS and NIMH funding has come through the BRAIN Initiative.
- The Department of Defense (DOD), including the Defense Advanced Research Projects Agency (DARPA) and United States Army.
- Private Foundations, including the Chan-Zuckerburg Initiative, the David and Lucile Packard Foundation, the Pew Charitable Trusts, the Sontag Foundation, the Dreyfus Foundation, the W.M. Keck Foundation and the Burroughs Wellcome Fund.
- Caltech also offers small grants for pilot funding as a research support mechanism.
Research Details
Who are your team members?
Our research laboratory currently includes nine postdoctoral scholars, 15 graduate students, four research staff, and seven undergraduate students. These individuals, and our lab alumni, are listed on our webpage.
Who are your internal and external collaborators?
Caltech is well known for its interdisciplinary collaborations, and that is a major reason that I came here. It also has a small faculty of around 300 people who really get to know each other and each other’s science. Our current internal collaborations related to ultrasound include the following investigators:
- Richard Andersen, PhD (Neuroscience): How use functional ultrasound readouts from the brain as a brain-machine interface. See Reading Minds with Ultrasound: Caltech’s New Brain–Machine Interface on SciTechDaily and Ultrasound Reads Monkey Brains, Opening New Way to Control Machines with Thought in Science Magazine.
- Doris Tsao, PhD (Neuroscience/Biology and Biological Engineering): How acoustically targeted chemogenetics can work in nonhuman primates.
- Michael Ortiz, PhD (Aeronautical and Mechanical Engineering): How to mathematically describe viscoelastic materials with solid-like and fluid-like properties. This work helps us understand how ultrasound interacts with the brain and with brain cancer.
- Shinsuke (Shin) Shimojo, PhD (Cognitive Neuroscience): How to understand sensory phenomena related to neurostimulation.
- Viviana Gradinaru, PhD (Neuroscience): How to enhance viral vectors for delivery into the brain.
- Michael Tyszka, PhD (Medical Physics): How to guide our focused ultrasound applications with MRI.
Our current external collaborators related to ultrasound include the following investigators:
- Mickäel Tanter, PhD, Physics for Medicine Paris: Hemodynamic functional ultrasound
- Charles Caskey, PhD, Vanderbilt University: Enhanced focused ultrasound targeting
- Stuart Foster, PhD, University of Toronto: was instrumental in helping us launch our work with gas vesicles
- Jinwoo Cheon, PhD, Institute for Basic Sciences, Yonsei University (Korea): combining ultraosound with magnetism
- Charles Liu, MD, PhD, University of Southern California: functional ultrasound brain-machine interfaces in humans
- Darrin Lee, MD, PhD, University of Southern California: acoustically targeted chemogenetics for neurological disease
- Inge Hermann, PhD, ETH Zürich: ultrasound monitoring of tissue adhesives
- James Friend, UCSD: mechanics of gas vesicles
- Ronny Drapkin, MD, PhD, University of Pennsylvania: cell-based diagnosis and therapy in ovarian cancer
- We have also sent gas vesicles, genetic constructs, and engineered cells to dozens of laboratories around the world directly from Caltech or through the Addgene repository.
What are your greatest achievements? Any major disappointments?
We’re still at a pretty early stage, but I am excited about several important first steps. One of the most important was getting mammalian cells to express gas vesicles—giving us the ability to interact with these cells through imaging and actuate them for effects like cavitation. This has opened up so many possibilities for us and others to pursue. I am also excited about the progress we have made with thermally controlled cell therapies in cancer and demonstrating how well acoustically targeted chemogenetics can work to control brain circuits. Of course, I wish we were moving even faster. I also wish more people in the ultrasound field would explore synthetic biology approaches. It’s not that hard, and my group is always happy to share published reagents and teach people. Most of our genetic reagents are freely available via Addgene and have been shipped all over the world.
What do you see as impediments to your success?
Of course, we could always use more funding. We are lucky to have excellent people and infrastructure at Caltech, but like almost everyone else we rely on external funding sources. We also need great people who are excited about our vision. I always enjoy hearing from postdoctoral candidates.
What is on your research wish list?
I would like to collaborate with more biologists and clinicians who want to apply the technologies we are developing to important problems. These could be established investigators or postdocs coming from a biology or medicine background who want to spin up a new and exciting direction in my lab.
Has the Foundation played a role in your work?
I have enjoyed interacting with and learning from the Foundation’s scientific team members, but otherwise the role has been small. I suspect the Foundation will at some point want to get more involved in synthetic biology as cell-based therapies, including gene editing, become a larger part of medicine. Focused ultrasound will have a role to play in this field. Ultrasound is one of the few good ways to achieve noninvasive spatial targeting.
Looking ahead, what comes next?
We would like to collaborate with disease-specific foundations and researchers, especially clinical researchers. Although Caltech does not have a medical center, we have opportunities to work with clinicians and researchers at the University of California, Los Angeles, the University of Southern California, and City of Hope Cancer Center. We would also like to build connections with developers of clinical instruments.
Past Coverage
Meeting Report: Focused Ultrasound Neuromodulation Symposium October 2019
Science News Top 10 Scientists to Watch September 2020
Videos
Veritasium: How Ultrasound Can Deactivate Parts of the Brain